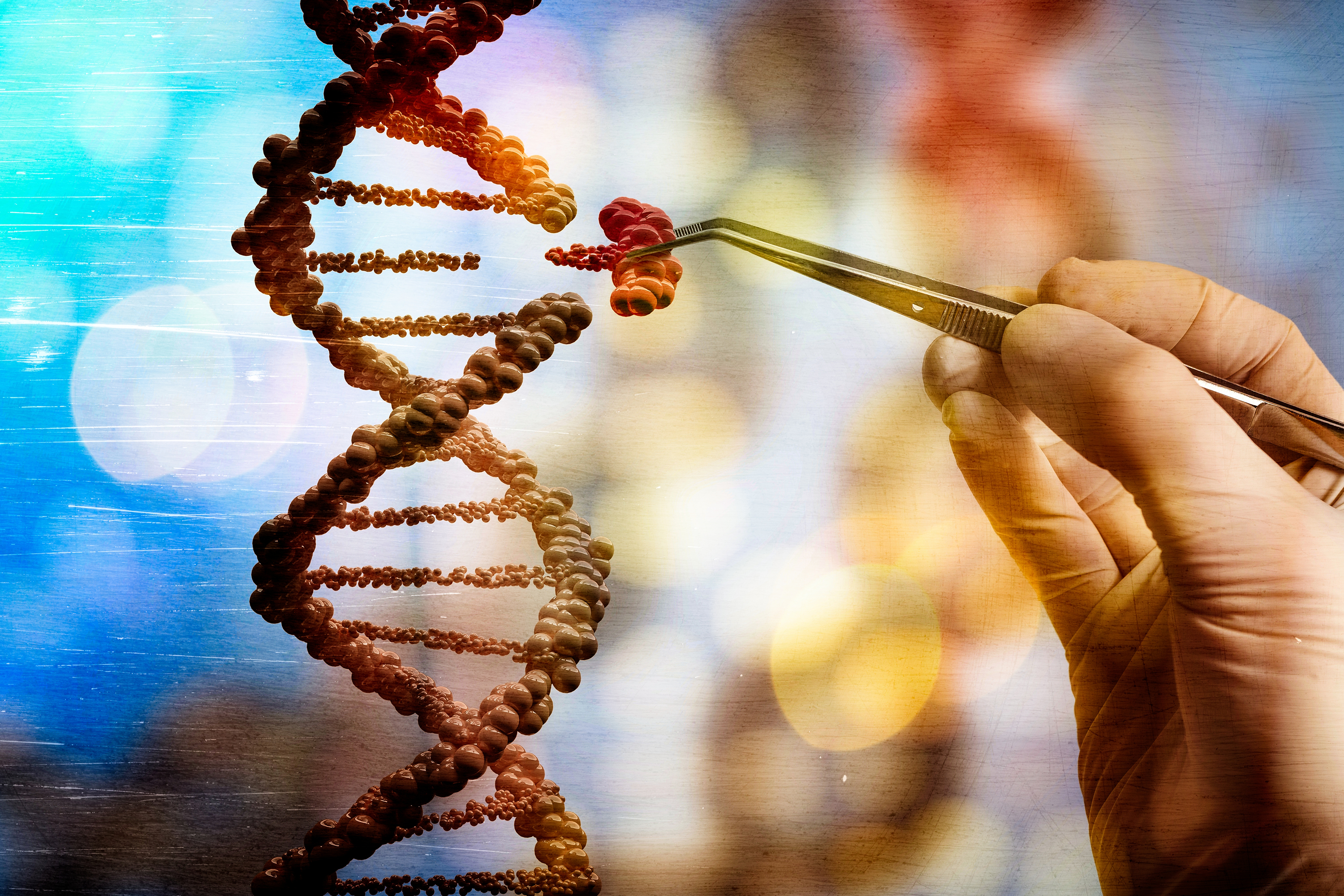
Genetic engineering is a field that has been rapidly advancing in recent years, with the potential to revolutionize healthcare, agriculture, and more. One of the most exciting developments in genetic engineering is CRISPR, a revolutionary gene-editing technology that has been described as a "genetic scalpel." With the ability to make precise and targeted changes to DNA, CRISPR has the potential to cure genetic diseases, create new medicines, and even improve the flavor and nutritional content of crops. In this blog post, we will explore the science behind CRISPR and genetic engineering, the potential applications of this technology, the ethical and regulatory challenges it poses, and the latest advances in the field. Whether you are a scientist, a student, or just someone interested in the future of biotechnology, this post will provide you with a comprehensive overview of CRISPR and genetic engineering. So, buckle up, and let's dive into the world of genetic engineering!
History of CRISPR
The history of CRISPR as a gene-editing tool begins with the discovery of the CRISPR system in bacteria in the early 1990s. CRISPR stands for "clustered regularly interspaced short palindromic repeats," which refers to the unique repeating pattern of DNA sequences found in the bacterial genome.In the early 2000s, scientists discovered that the CRISPR system could be reprogrammed to target specific genes in other organisms, including humans. However, it wasn't until 2012 that the major breakthrough came when Jennifer Doudna and Emmanuelle Charpentier published a paper in the journal Science showing that CRISPR could be used to make precise cuts in DNA in a test tube.
Their work showed that by combining the guide RNA with the Cas9 protein, they could create a molecular complex that could recognize and cut a specific DNA sequence. This opened up the possibility of using CRISPR to edit genes in living cells and organisms.
Since then, CRISPR has been rapidly adopted by scientists around the world as a powerful tool for gene editing. It has been used to edit genes in a wide range of organisms, from bacteria to mice to humans.
In 2015, the Broad Institute and the University of California Berkeley filed competing patent applications for the use of CRISPR in gene editing. The patent dispute was finally settled in 2017, with the US Patent and Trademark Office ruling in favor of the Broad Institute, which claimed the invention of using CRISPR-Cas9 for gene editing in eukaryotic cells.
How it works
The CRISPR-Cas system is a powerful tool for editing DNA that has been adapted from a bacterial defense mechanism against viruses. In bacteria, the CRISPR-Cas system provides a way to store and use genetic information to target and destroy invading viruses. Scientists have harnessed this system to create a powerful gene-editing technology that can be used to modify DNA in living cells.The CRISPR-Cas system works in three main steps: (1) recognition, (2) cleavage, and (3) repair.
Recognition: The first step in the CRISPR-Cas system is to identify the specific target DNA sequence that needs to be edited. This is done by designing a guide RNA (gRNA) that is complementary to the target DNA sequence. The gRNA is a small RNA molecule that is able to bind to the target DNA sequence through base-pairing interactions.
Cleavage: Once the gRNA has identified the target DNA sequence, it recruits the Cas protein to the site. The Cas protein is an enzyme that is able to cut DNA. The gRNA and Cas protein form a complex that binds to the target DNA sequence. Once the complex is bound to the DNA, the Cas protein cuts the double-stranded DNA at the target site. This creates a double-stranded break in the DNA.
Repair: Once the DNA is cut, the cell's natural DNA repair mechanisms are triggered to repair the break. There are two main mechanisms of DNA repair: non-homologous end joining (NHEJ) and homology-directed repair (HDR).
NHEJ is an error-prone repair mechanism that simply rejoins the two ends of the cut DNA molecule. This often results in small insertions or deletions (indels) at the cut site, which can cause frameshift mutations or disrupt gene function.
HDR, on the other hand, is a more precise repair mechanism that uses a template DNA molecule to repair the cut site. If a DNA template is provided that matches the sequence of the DNA adjacent to the cut site, the cell's repair mechanisms will use this template to repair the break, incorporating the new genetic material into the genome.
One way to use CRISPR is to introduce new genetic material into the genome by providing a DNA template that matches the sequence of the DNA adjacent to the cut site. Another way to use CRISPR is to disable or remove a specific gene by cutting the DNA at a specific site, causing the cell's natural repair mechanisms to either introduce errors that disrupt the function of the gene or remove the gene entirely.
CRISPR technology has revolutionized the field of gene editing, making it much easier and more precise to edit the genome than previous methods. However, it is still a complex and evolving technology that requires careful design and execution to achieve the desired outcome without causing unintended consequences.
Basic Research: CRISPR is a powerful tool for studying gene function and regulation. Scientists can use CRISPR to selectively edit genes in cells or animals and then observe the effects on cellular or physiological processes. This can help to identify the roles of specific genes in health and disease.
Biotechnology: CRISPR has many potential applications in biotechnology, including the production of genetically modified crops, animals, and microorganisms. By using CRISPR to selectively edit genes, scientists can create organisms with specific traits, such as disease resistance, increased productivity, or improved nutritional content.
Human Health: CRISPR has the potential to revolutionize medicine by enabling the correction of genetic mutations that cause disease. For example, in 2019, a patient with sickle cell disease was treated with CRISPR-edited cells in a clinical trial, with promising results. CRISPR could also be used to treat genetic disorders such as cystic fibrosis, Huntington's disease, and muscular dystrophy.
Disease Modeling: CRISPR can be used to create animal models of human diseases, allowing researchers to study the underlying mechanisms of disease and test potential therapies. For example, CRISPR has been used to create animal models of cancer, Alzheimer's disease, and muscular dystrophy.
Environmental Applications: CRISPR could be used to help address environmental challenges, such as climate change, by creating crops that are more resilient to drought or pests. CRISPR could also be used to engineer microorganisms that can break down pollutants in the environment.
CRISPR has the potential to have a significant impact on many different fields, from basic research to biotechnology and medicine. However, it is important to approach the use of CRISPR with caution and care to ensure that the technology is used ethically and responsibly.
CRISPR technology raises important ethical considerations around the use of gene editing. Some of the key debates around CRISPR include the potential for unintended consequences, the risk of creating unintended mutations or side effects, and the potential for eugenics or exacerbating social inequality. Additionally, the use of CRISPR in human embryos or reproductive cells raises ethical concerns about the creation of genetically modified humans.
Regulatory frameworks for genetic engineering vary between countries, with some countries having more strict regulations than others. National Health Institutions provide guidelines for research involving human subjects. However, there is an ongoing debate about whether current regulations are sufficient to address the potential risks and benefits of CRISPR, particularly in the context of human gene editing. Some experts have called for more robust oversight and regulation of CRISPR research and applications.
Myth: CRISPR can be used to create "designer babies".
Fact: While CRISPR has the potential to edit human embryos, it is currently illegal in many countries and is considered unethical by many scientists and policymakers. Additionally, the technology is still in its early stages and there are significant technical and ethical challenges that would need to be overcome before it could be used for this purpose
Myth: CRISPR can completely eradicate genetic diseases.
Fact: While CRISPR has shown promise in correcting genetic mutations, it is not a cure-all for genetic diseases. There are many complex genetic diseases that are caused by multiple genes and environmental factors, and it is unlikely that CRISPR will be able to completely eradicate all of these diseases.
Myth: CRISPR is not precise and can cause unintended mutations.
Fact: While CRISPR can cause unintended mutations, it is a highly precise tool when used correctly. Scientists use multiple safeguards to ensure that CRISPR only targets the desired genes and does not cause unintended mutations.
Myth: Genetic engineering is unnatural and dangerous.
Fact: Genetic engineering is a natural process that has been occurring for millions of years through evolution. Additionally, genetic engineering has the potential to benefit society through the development of new therapies and treatments.
Myth: CRISPR is easy to use and anyone can do it.
Fact: While CRISPR is a powerful tool, it requires significant technical expertise and resources to use effectively. It is not a simple or easy process and should only be attempted by trained scientists in a laboratory setting.
Delivery: One of the biggest challenges of using CRISPR is delivering it to the right cells or tissues. While CRISPR can target specific genes, getting it to the right cells or tissues can be difficult. Researchers are exploring various methods of delivery, such as viral vectors and nanoparticles, to improve the efficiency of CRISPR delivery.
Off-target effects: Another challenge of CRISPR is the risk of unintended effects on non-targeted genes. Researchers are working on improving the specificity of CRISPR by designing guide RNAs that are more specific to the target gene and developing new CRISPR systems that are more precise.
Ethics: The use of CRISPR raises important ethical questions, particularly in the context of human gene editing. Researchers and policymakers are working to develop ethical guidelines and regulations to ensure that the technology is used safely and responsibly.
Intellectual property: The development of CRISPR technology has led to a complicated intellectual property landscape, with multiple parties claiming ownership of key patents. This has led to legal disputes that could potentially impact the development and use of CRISPR in research and medicine.
Funding: Finally, the development and implementation of CRISPR technology require significant funding and resources. Researchers and institutions are working to secure funding and collaborations to continue advancing the technology and translating it into practical applications.
In conclusion, CRISPR is a powerful tool that has revolutionized genetic engineering and has the potential to transform medicine, agriculture, and many other fields. While CRISPR has many potential applications, it also raises important ethical, legal, and practical challenges that need to be addressed before it can be widely implemented. As scientists, policymakers, and the general public continue to grapple with these challenges, it's important to stay informed about the latest developments in CRISPR research and to engage in thoughtful discussions about the risks and benefits of this technology. With careful attention to safety, ethics, and regulatory frameworks, CRISPR could become an invaluable tool for addressing some of the most pressing challenges facing society today.
Cleavage: Once the gRNA has identified the target DNA sequence, it recruits the Cas protein to the site. The Cas protein is an enzyme that is able to cut DNA. The gRNA and Cas protein form a complex that binds to the target DNA sequence. Once the complex is bound to the DNA, the Cas protein cuts the double-stranded DNA at the target site. This creates a double-stranded break in the DNA.
Repair: Once the DNA is cut, the cell's natural DNA repair mechanisms are triggered to repair the break. There are two main mechanisms of DNA repair: non-homologous end joining (NHEJ) and homology-directed repair (HDR).
NHEJ is an error-prone repair mechanism that simply rejoins the two ends of the cut DNA molecule. This often results in small insertions or deletions (indels) at the cut site, which can cause frameshift mutations or disrupt gene function.
HDR, on the other hand, is a more precise repair mechanism that uses a template DNA molecule to repair the cut site. If a DNA template is provided that matches the sequence of the DNA adjacent to the cut site, the cell's repair mechanisms will use this template to repair the break, incorporating the new genetic material into the genome.
One way to use CRISPR is to introduce new genetic material into the genome by providing a DNA template that matches the sequence of the DNA adjacent to the cut site. Another way to use CRISPR is to disable or remove a specific gene by cutting the DNA at a specific site, causing the cell's natural repair mechanisms to either introduce errors that disrupt the function of the gene or remove the gene entirely.
CRISPR technology has revolutionized the field of gene editing, making it much easier and more precise to edit the genome than previous methods. However, it is still a complex and evolving technology that requires careful design and execution to achieve the desired outcome without causing unintended consequences.
Applications of CRISPR
The potential applications and current uses of CRISPR are numerous and diverse, ranging from basic research to biotechnology and medicine. Here are some examples:Basic Research: CRISPR is a powerful tool for studying gene function and regulation. Scientists can use CRISPR to selectively edit genes in cells or animals and then observe the effects on cellular or physiological processes. This can help to identify the roles of specific genes in health and disease.
Biotechnology: CRISPR has many potential applications in biotechnology, including the production of genetically modified crops, animals, and microorganisms. By using CRISPR to selectively edit genes, scientists can create organisms with specific traits, such as disease resistance, increased productivity, or improved nutritional content.
Human Health: CRISPR has the potential to revolutionize medicine by enabling the correction of genetic mutations that cause disease. For example, in 2019, a patient with sickle cell disease was treated with CRISPR-edited cells in a clinical trial, with promising results. CRISPR could also be used to treat genetic disorders such as cystic fibrosis, Huntington's disease, and muscular dystrophy.
Disease Modeling: CRISPR can be used to create animal models of human diseases, allowing researchers to study the underlying mechanisms of disease and test potential therapies. For example, CRISPR has been used to create animal models of cancer, Alzheimer's disease, and muscular dystrophy.
Environmental Applications: CRISPR could be used to help address environmental challenges, such as climate change, by creating crops that are more resilient to drought or pests. CRISPR could also be used to engineer microorganisms that can break down pollutants in the environment.
CRISPR has the potential to have a significant impact on many different fields, from basic research to biotechnology and medicine. However, it is important to approach the use of CRISPR with caution and care to ensure that the technology is used ethically and responsibly.
CRISPR technology raises important ethical considerations around the use of gene editing. Some of the key debates around CRISPR include the potential for unintended consequences, the risk of creating unintended mutations or side effects, and the potential for eugenics or exacerbating social inequality. Additionally, the use of CRISPR in human embryos or reproductive cells raises ethical concerns about the creation of genetically modified humans.
Regulatory frameworks for genetic engineering vary between countries, with some countries having more strict regulations than others. National Health Institutions provide guidelines for research involving human subjects. However, there is an ongoing debate about whether current regulations are sufficient to address the potential risks and benefits of CRISPR, particularly in the context of human gene editing. Some experts have called for more robust oversight and regulation of CRISPR research and applications.
Misconceptions around CRISPR
There are several common misconceptions about CRISPR and genetic engineering that can lead to confusion and misunderstanding. Here are some of the most common myths and the accurate information that can help readers understand the facts:Myth: CRISPR can be used to create "designer babies".
Fact: While CRISPR has the potential to edit human embryos, it is currently illegal in many countries and is considered unethical by many scientists and policymakers. Additionally, the technology is still in its early stages and there are significant technical and ethical challenges that would need to be overcome before it could be used for this purpose
Myth: CRISPR can completely eradicate genetic diseases.
Fact: While CRISPR has shown promise in correcting genetic mutations, it is not a cure-all for genetic diseases. There are many complex genetic diseases that are caused by multiple genes and environmental factors, and it is unlikely that CRISPR will be able to completely eradicate all of these diseases.
Myth: CRISPR is not precise and can cause unintended mutations.
Fact: While CRISPR can cause unintended mutations, it is a highly precise tool when used correctly. Scientists use multiple safeguards to ensure that CRISPR only targets the desired genes and does not cause unintended mutations.
Myth: Genetic engineering is unnatural and dangerous.
Fact: Genetic engineering is a natural process that has been occurring for millions of years through evolution. Additionally, genetic engineering has the potential to benefit society through the development of new therapies and treatments.
Myth: CRISPR is easy to use and anyone can do it.
Fact: While CRISPR is a powerful tool, it requires significant technical expertise and resources to use effectively. It is not a simple or easy process and should only be attempted by trained scientists in a laboratory setting.
Challenges of CRISPR
While CRISPR is a promising technology, there are several practical challenges that need to be overcome before it can become a practical tool for scientists and doctors. Here are some of the key challenges and how researchers are currently addressing them:Delivery: One of the biggest challenges of using CRISPR is delivering it to the right cells or tissues. While CRISPR can target specific genes, getting it to the right cells or tissues can be difficult. Researchers are exploring various methods of delivery, such as viral vectors and nanoparticles, to improve the efficiency of CRISPR delivery.
Off-target effects: Another challenge of CRISPR is the risk of unintended effects on non-targeted genes. Researchers are working on improving the specificity of CRISPR by designing guide RNAs that are more specific to the target gene and developing new CRISPR systems that are more precise.
Ethics: The use of CRISPR raises important ethical questions, particularly in the context of human gene editing. Researchers and policymakers are working to develop ethical guidelines and regulations to ensure that the technology is used safely and responsibly.
Intellectual property: The development of CRISPR technology has led to a complicated intellectual property landscape, with multiple parties claiming ownership of key patents. This has led to legal disputes that could potentially impact the development and use of CRISPR in research and medicine.
Funding: Finally, the development and implementation of CRISPR technology require significant funding and resources. Researchers and institutions are working to secure funding and collaborations to continue advancing the technology and translating it into practical applications.
In conclusion, CRISPR is a powerful tool that has revolutionized genetic engineering and has the potential to transform medicine, agriculture, and many other fields. While CRISPR has many potential applications, it also raises important ethical, legal, and practical challenges that need to be addressed before it can be widely implemented. As scientists, policymakers, and the general public continue to grapple with these challenges, it's important to stay informed about the latest developments in CRISPR research and to engage in thoughtful discussions about the risks and benefits of this technology. With careful attention to safety, ethics, and regulatory frameworks, CRISPR could become an invaluable tool for addressing some of the most pressing challenges facing society today.
Latest Development in CRISPR